Contact Us
- Address: Réservations par internet seulement
- Email: infoSPAMFILTER@spaceobs.com
- Phone: No telephone
- Cellphone: No telephone
- Website: www.spaceobs.com
Next moons
22-12-2024 ![]() | 30-12-2024 ![]() |
06-01-2025 ![]() | 13-01-2025 ![]() |
Sponsors
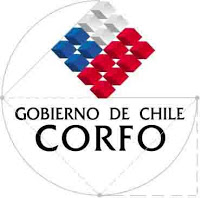
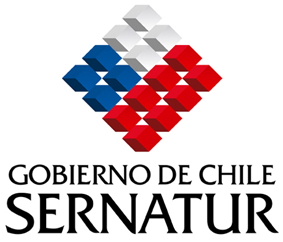
I really don't have the time to maintain several pages in various languages. So please refer to the page in french, and then google translate it. Really sorry.
22-12-2024 ![]() | 30-12-2024 ![]() |
06-01-2025 ![]() | 13-01-2025 ![]() |